Highlights
TMT causes cognitive deficits and impaired working and avoidance memory.
TMT intoxication disturbs antioxidant system and BDNF expression in hippocampus.
Swimming exercise and GA ameliorates TMT-induced cognitive deficits by reducing oxidative stress and BDNF expression in hippocampus.
The use of this supplement can be useful for athletes, ordinary people, heart patient, and sports coaches.
Introduction
Trimethyltin (TMT) is an organotin with a severe neurotoxic property which has become an environmental pollutant because of its abundant use in plastic industry, silicon products, food packaging, and pesticides
(1). This mixture sometimes causes severe poisoning in animals and humans by entering drinking water of houses, aquatic and marine environments
(2). Using TMT as a tool of neurodegenerative analysis in laboratory studies has an interesting application in evaluating molecular events along with hippocampus neuron damages in cognitive disorders of animal models
(3). In human, TMT intoxication results in cerebellar limbic syndrome which is associated with disorder in memory, confusion, seizure, amnesia, insomnia, and depression
(4). TMT damage in hippocampus pyramidal cells and dentate neurons can occur 2-3 days after intoxication and this damage is rooted in severe stimulation of microglia and astroglia in hippocampus
(5). The relations of hippocampus and cognitive disorder damages (memory and cognitive disorders) because of TMT causes that it is used as a model to study Alzheimer’s disease
(6).
TMT induced hippocampal degeneration can occur because of neurological inflammation, stimulatory toxicity due to calcium overload, mitochondria dysfunction and oxidative stress
(7). However, apoptosis mechanism due to TMT is remain unknown. In the meantime, stimulatory toxicity dependent to glutathione has been raised as a main factor among other mechanisms
(8). Studies done on TMT neurological degeneration have shown that intoxication with this compound is associated with decreased expression of B-cell lymphoma 2 (Bcl2), BCL2 Like 1 (Bcl2L1) and brain-derived neurotrophic factor (BDNF) in the hippocampus
(9). In hippocampus oxidative stress, reactive oxygen species (ROS), reactive nitrogen increase (RNS), and lipid peroxidation are produced
(10). Therefore, using antioxidants such as GA can be effective in treatment of neural damages due to TMT and ameliorating of cognitive disorders.
GA is a polyphenolic compound and the product of hydrolysis of tannins in plants, which has antibacterial, antifungal, antiviral, anti-inflammatory and antioxidant activity by inhibiting the activity of tyrosine and helps the body cells to protect against oxidative damage
(11). This compound also has anti-cancer properties and is used as a medicine in the treatment of diabetes
(12). GA can easily cross the blood-brain barrier, improve neuronal function in cognitive disorder in animal models, and protect against nerve cell death due to amyloid β (Aβ) peptides
(13).
Various evidence has confirmed the role of regular exercise and physical activity in mental and physical health. Exercise leads to an increase in the phenomenon of long-term reinforcement in dentate gyrus of rat hippocampal formations as well as neurogenesis and improved performance in behavioral tests of learning and memory
(14). The beneficial effects of exercise have been attributed to increased levels of neurotrophins, especially BDNF, in the hippocampus and limbic system
(15). Reinforcement of the brain's antioxidant defense system and increasing the activity of antioxidant enzymes due to adaptation to exercise after oxidative stress, reduces damage to the brain
(16). It is important to understand the mechanisms associated with physical activity in hippocampus damage despite the effective role of physical activity in cognitive disorders control and the effectiveness of exercise on brain health. Therefore, the purpose of the present study is to evaluate the effect of GA and swimming exercise on memory and learning deficits, oxidative stress parameters and BDNF hippocampus expression in the TMT model of Alzheimer's disease (AD).
Materials and Methods
Animals, grouping and model induction
In this developmental study with experimental research method, 40 male Wistar rats with an average weight of 210 ± 10 g and approximately 2.5 months were used. These animals were prepared from the Animal Breeding and Reproduction Center of Shiraz University of Medical Sciences and transferred to the animal room of Islamic Azad University, Shiraz Branch. In order to adapt animals to the new environment, the experiments were performed one week after the animals’ adaptation. The animals were kept under standard conditions of 25±2°C, relative humidity of 50±10% and a 12-hour lightness / darkness cycle (6 a.m. to 6 p.m.). In this study, food for laboratory rats with standard formulation (110 Fars Livestock and Poultry Feed Production Company) was used, which was freely available to animals (ad libitum) along with tap water. Ethical principles were observed in accordance with international law and the rules of the Ethics Committee of Laboratory Animals of Islamic Azad University, Shiraz Branch (Ethics Code: IR. IAUSHIRAZ.1399.18.45).
Rats were randomly divided into 5 groups of eight which includes healthy control group, TMT+NS (receiver of TMT and normal saline as GA solvent), TMT+GA200 (receiver of TMT and 200 mg/kg of GA), TMT+EX (receiver of TMT and eight weeks of swimming exercise), TMT+ GA200+EX (receiver of TMT, 200 mg/kg of GA and 8 weeks of swimming exercise). To induce TMT intoxication, a dose of 8 mg/kg body weight of TMT (Sigma, Germany) was injected intraperitoneally with normal saline
(17). 24 hours after TMT injection, groups receiving GA (Sigma, Germany) received a dose of 200 mg/kg orally (gavage) for 8 weeks and 3 days a week (days in which swimming exercise weren’t done) in the early morning hours.
Swimming exercise protocol
Exercise protocol included 8 weeks of exercise in circular swimming pool specified for rodents with 1-meter diameter, 50 cm height and the water temperature of 25 ±2 °C for 20 minutes every session and 3 sessions per week (days in which GA wasn’t administrated). Animals entered the pool 1 minute before exercise to be familiar with the environment and warm up. In the main phase of exercise, they were released from a fixed part of the pool and swam to the hidden platform. The circular hidden platform is 10 cm in diameter and made of transparent Plexiglas, 1 cm under water. As soon as it reached the platform, the position of the platform was changed so that the animal could continue swimming
(18). This was done consistently for all animals. After exercise, the animals were dried and transferred to their cages. During the week before the main exercise, the animals practiced swimming for 8 minutes, adding 2 minutes to the swimming time per day until it reached 20 minutes at the end of the week. Swimming speed was determined in all rats using the tracking software. The average swimming speed was the same in all groups (results haven’t not given).
Assessment of passive avoidance memory
The test was performed using a shuttle box that consists of two light and dark room of equal size, which are connected by a guillotine door. Steel rods connected to the electroshock device were at the floor of the room. During the adaptation phase, the animal entered the light room and was allowed to enter the dark room itself. The latency time to the dark room and the duration of staying in the dark and light rooms were recorded for 300 seconds. In the training phase, after the animal’s first entrance to the dark room, a guillotine door was immediately closed between the two rooms and a 2 mA, 50 Hz shock was inserted into the animal through metal rods on the floor of the dark room for 2 seconds. Then the door of the container was removed so that the animal can enter the light room itself. This step was performed one day after the acquisition step. In the test phase, 24 and 48 hours after the shock, the animal entered the light room again and the latency time to the dark room and the duration of staying in the dark room were recorded for 300 seconds. In this phase, the animal did not receive any shocks after entering the dark room
(19).
Assessment of working memory: Y maze test
This test was used to evaluate working memory by assessing the percent of alteration behaviors. The Y maze consisted of three arms with dimensions of 60×15×15 cm each, connected by a triangular central area. First, each animal was placed at the end of an arm, allowing the animal to have free access to the other arms of the maze over a period of 5 minutes. Rodents typically preferred to check the new arm and weren’t reluctant to return to the arm they had previously seen, unless they had forgotten it. Animal’s entrance into each arm was recorded by observation. Animal’s entrance into the arm was considered when the animal’s hind limbs were completely inside the arm, the alteration behaviors were considered as successful and consecutive (serial) entries into all the arms in overlapping 3 sets. Thus, the observed alteration percentage was calculated multiplied by 100 to the maximum rotation (total number of inserted arms)
(20).
Biochemical Analysis
After behavioral test and at the end of the eight weeks training, animals in each group were anesthetized with a lethal dose of chloroform and the animal was beheaded immediately with a rodent guillotine. After separating the brain from the skull, the brain was quickly placed on ice. The hippocampus was carefully separated from the rest parts under a stereoscope (Olympus, Japan). After washing with saline solution and Tris buffer (Sigma, Germany), hippocampal tissue was homogenized for 5 minutes with the homogenizer (MTOPS, Korea) at 5000 doses per minute. The homogenized solution was centrifuged and phenyl-methyl sulfonyl fluoride 0.5 mM solution (Sigma, Germany) was used as a protease inhibitor. After centrifugation, the supernatant was removed with a sampler and investigated to evaluate oxidative stress factors
(21).
The ELISA method and kit of ZellBio company (ZellBio, Germany) were used to measure the hippocampal level of CAT and TAC. Also, the tissue content of MDA was evaluated based on reaction with thiobarbituric acid (Merck, Germany), absorption measurement by spectroscopy and comparison of absorption with standard curve. BDNF tissue was measured by ELISA kit (Rat BDNF PicokineTM, Boster, China). The initial content of tissue and dilution of tissue extract were considered the same in all samples according to the protocol of ELISA kits.
Statistical analysis
The statistical analysis between groups was done by SPSS software version 22. Data were reported in the form of mean ± standard deviation. For the normal distribution of data, Shapiro-Wilk test was used. Also, an independent t-test, two-way analysis of variance and Bonferroni post hoc test were used to determine the significant difference between groups. A value of p˂0.05 was considered statistically significant
Result
Working memory
The results of data analysis of groups showed that the percentage of alteration behaviors (working memory) in TMT+NS group compared to control groups had a significant decrease (p<0.001). On one hand, GA treatment causes the amelioration of working memory and significant increase compared to TMT+NS group (p<0.01). Also, swimming exercise increases significantly in working memory compared to TMT+NS group (p<0.01). Although, there wasn’t any difference between TMT+GA200 and TMT+EX groups, however; alteration behaviors in TMT+GA200+EX group had a significant increase compared to TMT+GA200 and TMT+EX groups (p<0.05,
figure 1).
In the investigation of the effect of treatment, the results of two-way variance test and Bonferroni post hoc test showed that swimming exercise (p= 0.007) and Gallic acid administration (p< 0.001) had a significant effect on the increase of working memory (alteration behaviors) in the cognitive deficit induced TMT in rats. Swimming exercise and GA treatment were effective in increasing the working memory of animals in TMT intoxication model (p< 0.05).

Figure 1. Comparison of mean ± standard deviation of frequency percentage of alteration behaviors in different groups. The results show that there was a significant difference between the control group and TMT+NS, TMT+GA200 and TMT+EX groups (***p <0.001 and **p <0.01). In TMT+GA200, TMT+EX and TMT+GA200+EX groups, a significant increase was observed compared to TMT+NS group (***p <0.001 and **p <0.01). Also, a significant increase was observed in TMT+GA200+EX group compared to TMT + EX ($p <0.05) and TMT+GA200 (#p <0.05) groups.
Passive avoidance memory
In shuttle box test, latency to the dark room before shock induction (acquisition phase), a significant difference wasn’t seen between the groups
(figure 2). 24 hours after the shock induction, latency to the dark room showed there was a significant difference between control groups and other groups (TMT+NS, TMT+GA200, TMT+EX, and TMT+GA200+EX groups (p<0.001). in all treatment groups, there was a significant decrease in the duration of latency to the dark room compared to control group. Also, there was a significant increase in latency to the dark room of TMT+GA200 (p<0.01) TMT+EX (p<0.05) and TMT+GA200+EX groups (p<0.01) compared to TMT+NS (figure2). There was a significant decrease in the duration of latency to the dark room 48 hours after the shock induction of TMT+NS, TMT+GA200, TMT+EX, and TMT+GA200+EX groups (p<0.001). also there was a significant increase in TMT+GA200, TMT+EX, TMT+GA200+EX groups compared to TMT+NS group (p<0.01).
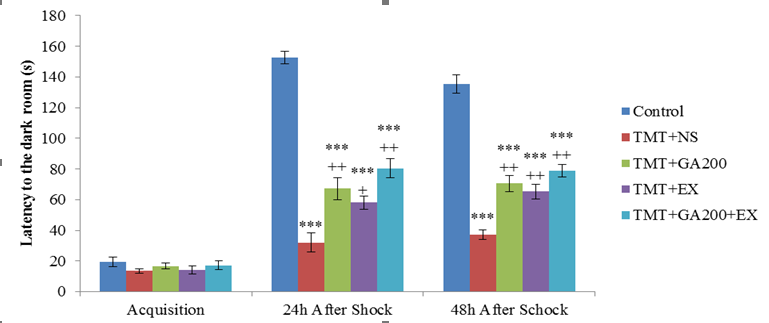
Figure 2. Comparison of the mean ± standard deviation of the latency to the dark container. In the acquisition phase, no significant differences were observed between the groups. 24 and 48 hours after shock induction, there was a significant difference between the control group and TMT+NS, TMT+GA200, TMT+EX and TMT+GA200+EX groups (***p<0.0001). Also, there was a significant difference between TMT+NS group and TMT+GA200, TMT+EX and TMT+GA200+EX groups at 24 and 48 hours after shock induction (++p<0.01 and +p<0.05)
In the assessment of mean of the existence time of the animals in the dark room, no significant difference was seen between the groups while after 24 hours of shock induction, this time increased significantly in TMT+NS (p<0.001), TMT+GA200, and TMT+EX (p<0.01) groups. In comparison to TMT+NS, TMT+GA200 and TMT+EX groups in p<0.01 level and TMT+GA200+EX group in p<0.001 level showed a significant difference. A significant difference was seen between TMT+GA200 and TMT+EX groups to TMT+GA200+EX group (p<0.05). The statistical analysis after 48 hours of shock induction showed that TMT+NS and TMT+GA200 had a significant increase in the time spent in dark room compared to control group (p<0.001). While no difference was seen between TMT+EX and TMT+GA200+EX groups compared to control group. On the other hand, in comparison to TMT+NS group, TMT+EX and TMT+GA200+EX had a significant decrease (p<0.01 and p<0.001, respectively). A significant difference was seen in TMT+GA200+EX group in comparison to TMT+EX and TMT+GA200 groups (respectively p<0.05 and p<0. 01) and also TMT+GA200 group with TMT+EX group (p<0.05,
figure3).

Figure 3. Comparison of the mean ± standard deviation of the time spent in the dark room. In the acquisition phase, no significant differences were observed between the groups. After the shock induction, there was a significant difference between the control group and TMT+NS, TMT+GA200, TMT+EX groups (***p<0.001, **p<0.01). On the other hand, TMT+NS group showed a significant difference with TMT+GA200, TMT+EX and TMT+GA200+EX groups after shock induction (++p<0.001, +p<0.01, +p<0.05). 24 hours after shock induction, TMT + GA200 + EX group had a significant decrease compared to TMT + GA200 ($p<0.05) and TMT+EX (#p<0.05) groups. 48 hours after shock induction, TMT+EX group showed a significant difference compared to TMT+GA200 and TMT+GA200+EX groups ($p <0.05 and $$p<0.01). The TMT+GA200 group showed a significant difference compared to the TMT+EX group (#p<0.05).
Assessment of hippocampal level of BDNF
TMT intoxication reduces BDNF protein expression obviously in rats’ hippocampus compared to control group, in a way that BNDF content was significantly lower in the hippocampus tissue extract of TMT+NS group than control group (p <0.001,
figure4). Also, the was a significant decrease in BDNF level of TMT+GA200 and TMT+EX groups compared to control group (p<0.01). However, GA treatment, swimming exercise and the interaction of these two treatments significantly increased the BDNF hippocampus compared to the TMT + NS group. In fact, there was a significant increase (p<0.001) in all three groups of TMT+GA200, TMT+EX and TMT+GA200+EX compared to TMT+NS group. There was a significant difference between TMT+GA200 and TMT+EX groups and TMT+GA200+EX group (p<0.05).
.png)
Figure 4. Comparison of mean ±standard deviation of hippocampal level of BDNF in different groups. There was a significant difference between the control group and TMT+NS, TMT+GA200 and TMT+EX groups (***p<0.001 and **p<0.01). Also, there was a significant difference between TMT+GA200, TMT+EX and TMT+GA200+EX groups with TMT+NS group (***p<0.001). TMT+GA200+EX group was significantly different from TMT + GA200 ($p<0.05) and TMT + EX (# p<0.05) groups.
Assessment of hippocampal level of CAT, TAC and MDA
The results showed that hippocampal level of CAT in TMT+NS, TMT+GA200 (p<0.001) and TMT+EX (p<0.01) groups had a significant decrease compared to control group. While by comparing to TMT+NS group, TMT+EX (p<0.01) and TMT+GA200+EX (p<0.001) had a significant increase in hippocampal level of CAT. TMT+EX and TMT+GA200+EX groups had more CAT compared to TMT+GA200 group (p<0.01 and p<0.001, respectively). Also, TMT+GA200+EX group had a significant increase compared to TMT+EX group (p<0.05).
In the assessment of TAC, all groups had a significant increase compared to control group. In fact, hippocampal content of TAC had significant decrease in TMT+NS, TMT+GA200, and TMT+EX groups in p<0.001 and TMT+GA200+EX group in p<0.01 compared to control group. Despite this, only TMT+GA200+EX group increased significantly than TMT+NS group (p<0.05). Also, there was seen a significant difference in TMT+GA200+EX group compared to TMT+GA200 and TMT+EX groups (p<0.05).

Figure 5. Comparison of mean ± standard deviation of hippocampal level of catalase (CAT), total antioxidant capacity (TAC) and malondialdehyde (MDA) in different groups. Compared with the control group, TMT+NS, TMT+GA200 and TMT+EX groups had a significant decrease in CAT and TMT+NS, TMT+GA200, TMT+EX and TMT+GA200+EX groups had a significant decrease in TAC. Also, a significant increase in MDA content was seen in the TMT+NS group compared to control group (***p<0.001, **p<0.01). Also, compared with TMT+NS group, there was a significant difference in TMT+EX and TMT+GA200+EX groups in CAT level and in TMT+GA200+EX group in TAC and in TMT+NS, TMT+GA200, TMT+EX and TMT+GA200+EX were seen in MDA content (+p<0.05, ++p<0.01, +++p<0.001). Compared with TMT+GA200 group, TMT+EX and TMT+GA200+EX groups showed a significant difference in CAT content and TMT+GA200+EX group in TAC ($p<0.05, $$p<0.01, $$$p<0.001). There was a significant difference in CAT and TAC content between TMT+EX group and TMT+GA200+EX group (#p<0.05).
Discussion
The results of the present study have shown that TMT intoxication causes a damage in working and passive avoidance memories. After TMT intoxication, the BDNF hippocampal expression decreased. CAT level and TAC had a significant decrease and the level of lipid peroxidation (hippocampal content of MDA) had a significant increase. Also, the increased activity of CAT antioxidant enzyme and TAC along with reduction of MDA and amelioration of working and passive avoidance memories after 8 weeks of swimming and GA treatment were observed in the studied groups.
TMT is a neurotoxic organotin that causes cognitive disorders, memory and learning loss in rats by selective neuronal death in the limbic system, particularly the hippocampus. Thus, it is an efficient model for investigating the behavioral changes depending on neurological analyzes such as hyperactivity, aggression and cognitive deficits and experimental models in AD-like studies
(3). Numerous evidence has shown that TMT alters the expression of effective neurotransmitters in memory such as acetylcholine and glutamate. TMT damages the hippocampal GABAergic system and reduces the desire to reabsorb this neurotransmitter
(10).
The previous studies have shown that TMT injection (8mg/kg) destroys hippocampal pyramidal cells, causes cognitive disorders, reduces BDNF values and increases inflammation in rats
(22). Neurotrophins are the most important factors in the proliferation, differentiation, maintenance, plasticity, survival and function of nerve cells. In the meantime, BDNF is an important member of polypeptide neurotrophins, a major regulator of axonal growth, neural differentiation, survival, and synaptic plasticity. Synaptic plasticity needs BDNF to improve the process of memory and learning. Therefore, it seems that the occurrence of premature memory deficit in AD has a direct relation with the reduction of BDNF and TrkB values as the main receptors at the cell surface, especially in the hippocampus
(23).
The increase of hippocampal level of BDNF along with decreased working memory disorder, improved avoidance memory and increased expression of antioxidant enzymes after eight weeks of swimming exercise were seen in the present study. Regarding the effect of physical activity on the BDNF signal, the researchers showed that 8 weeks of resistance exercise increased BDNF and TrkB in the hippocampus of adult male rats. Resistance exercise and the combination of resistance exercise and running are effectively associated with the increase in the amount of neurotrophins in the hippocampus, which improves the function of the BDNF and TrkB signal pathways after exercise and can improve cognitive functions in rats
(24). Although the exact causes of the effect of exercise on neurotrophic expression have not yet been identified, some of these effects appear to be related to reactive oxygen species (ROS) and changes in cell redox homeostasis. Following physical activity, increasing oxygen consumption increases the production of ROS to the point that it optimizes oxidation signals and regulates signaling pathways and gene expression in various tissues, including the brain and heart. This improves mitochondrial respiration, reduces lipid peroxidation and strengthens the antioxidant defense system in these tissues
(25). BDNF by connecting to the TrkB receptors activates protein kinase A (PKA), protein kinase C (PKC), mitogen-activated protein kinase (MAPK), and a transcriptional regulator called connective protein to the response element cAMP (CREB)
(26). Sport activities can affect synaptic plasticity in presynaptic and postsynaptic terminals by affecting BDNF expression. This mechanism is in the form of BDNF signal conduction through the TrkB receptor, whose expression is regulated by exercise
(27). It seems that long term potency (LTP) is increased by exercise in different areas of the hippocampus. As a result, exercise enhances memory and learning through mechanisms such as increased BDNF level as mediators of synaptic effects, neural connections, and neuroplasticity in the brain
(28). Also, exercise improves nerve cell function through cell proliferation in the hippocampus and apoptosis inhibition in the dentate gyrus of the hippocampus and synaptic space increase in different parts of the brain
(27). However, studies have shown that the type, intensity and duration of the exercise period are effective factors in neurotrophins changes
(29). Aerobic exercise increases protein expression associated with synaptic plasticity and the BDNF signal in the hippocampus of diabetic rats
(30). Although, in the present study, eight weeks of swimming exercise significantly improved working memory, another study showed that eight weeks of endurance exercise had no effects on working memory in the TMT model of AD, however; it improved avoidance memory
(31). Therefore, it can be said that the type and intensity of exercise are involved in the cellular mechanism to improve working memory and hippocampal function.
TMT induced oxidative stress and the role of herbal antioxidants led to the investigation of the role of GA. The present study showed the obvious increase of working memory, passive avoidance memory and decrease of lipid peroxidation following the treatment of GA, however; no obvious increase of hippocampal activity of CAT and TAC was seen. Despite the antidiabetic, anti-angiogenic and anti-tumor effects, acid can reduce myocardial infarction index and prevent oxidative damage to liver and kidney
(32). GA improves neural damages and cognitive disorders in animal models of AD
(33). Also, GA improves brain injury, defects in hippocampal LTP, and induced pathology after cerebral hypo-perfusion in rats
(34). Also, treatment with GA reduces the inflammation, improves brain electrophysiological function, and behavior in rats following a model of traumatic brain injury (TBI), possibly by reducing pre-inflammatory cytokines in the brain
(35). GA has a neuroprotective effect on LTP damage and memory associated with TBI by reducing cerebral lipid peroxidation and cerebral pre-inflammatory cytokines
(36). GA pretreatment corrects ischemia and cerebral reperfusion injury and stimulates the defense system antioxidant in the face of ischemia and reperfusion in rats
(37). Maya et.al., showed that GA and wedelolactone have neuroprotective effects on aluminum-induced neurodegeneration and do this by improving their antioxidant status, increasing BDNF expression, preventing glutamate excitotoxicity, inhibiting caspase-3 activity, and negative regulation of inflammatory cytokines
(38). GA has been shown to increase the expression of BDNF and its receptor (TrkB), in the hippocampus following mild chronic stress
(39) and improve the symptoms of inflammation in the brain by modifying lipid peroxidation and brain proteins
(40).
The results of the present study showed that 8 weeks of swimming exercise along with GA administration have more effects on the amelioration of working memory and passive avoidance memory, BDNF, CAT activity and TAC content in hippocampus than swimming exercise or GA treatment separately in rats following TMT intoxication. Despite finding studies in the assessment of the effect of GA or exercises on physiologic and cognitive parameters in various models of AD, there weren’t studies that investigate the effect of GA and exercises (swimming) simultaneously on cognitive performance and BDNF expression in rats suffering from AD. However, the previous studies investigated the effect of GA and swimming exercise separately, by reviewing the studies, we can conclude that swimming exercise and GA possibly improve learning and memory with the same effect mechanism.
Conclusion
The interactive effects of swimming exercise along with GA ameliorate cognitive functions in rats after TMT intoxication by increasing total antioxidant capacity and hippocampal activity of antioxidant enzymes, reducing lipid peroxidation and increasing hippocampal level of BDNF.
Acknowledgment
Authors would like to thank Dr. Habibollah Khodabandeh for the technical assistant.
Conflict of interests
The authors have no conflicts of interest to declare.
How to cite:
Rafiei S, Ravasi AA, Gaeini AA. The Effect of Eight-week Swimming Exercise and Gallic Acid on Hippocampal BDNF and Oxidative Stress Parameters in Trimethyltin Induced Cognitive deficits; Jorjani Biomedicine Journal. 2021; 9(1):55-68.
|
|